When the U.S. Department of Energy announced on December 13, 02022 that researchers at the National Ignition Facility (NIF) at the Lawrence Livermore National Laboratory had conducted the first ever controlled nuclear fusion experiment to achieve ignition, it marked a key milestone in the history of energy: the first time a human-caused fusion reaction had produced more energy than it consumed. The NIF’s fusion breakthrough was not a shocking, out-of-the-blue discovery, but the result of decades of diligent research and experimentation in the field.
In fact, the news of the NIF ignition came exactly two years to the month after Long Now Founder Danny Hillis forecasted it would in 02010. In Long Bet #605, Hillis predicted that “by December 31, 02020, synthetic solar energy (fusion) will have been shown to be technically feasible, by an experiment demonstrating a controlled fusion reaction producing more harnessable energy than was used to ignite it.” Hillis’ prediction, while premature in its timing, follows a long history of hope for human-harnessed fusion as a potent source of energy.
The earliest recorded scientific reference to fusion as a potential source of energy came more than a century ago. In 01920, Arthur Eddington, an early astrophysicist and popularizer of Einstein’s theory of General Relativity, gave a speech at the British Association for the Advancement of Science on "The Internal Constitution of the Stars." While Eddington himself did not know for certain the mechanics of how nuclear fusion powered the stars — no one would for more than a decade after — he speculated that “subatomic energy” must be the continual fuel for the sun and other stars. He even expressed that he and his fellow scientists “sometimes dream that man will one day learn how to release it and use it for his service,” noting that “the store is well-nigh inexhaustible, if only it could be tapped.”
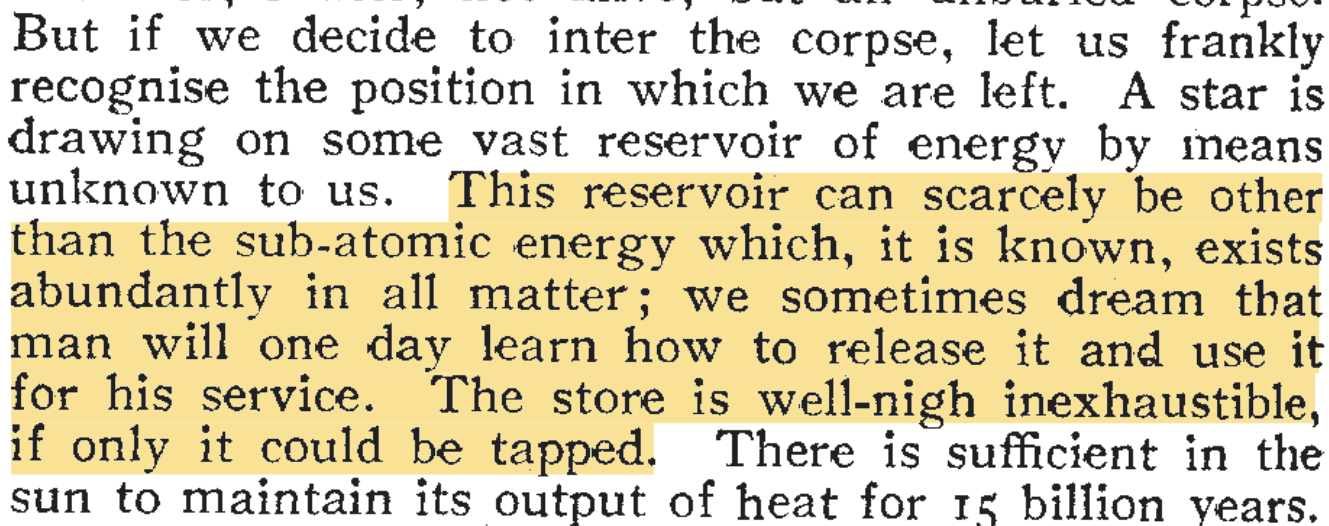
In the first half of the twentieth century, physicists sought to understand the basic mechanics of nuclear fusion, with the first patent for a fusion reactor being registered in 01946. In the 01950s, the search for nuclear fusion power began in earnest, with a number of promising experiments conducted in the United States, United Kingdom, and elsewhere. In 01954, U.S. Atomic Energy Commission Chairman Lewis Strauss gave a speech proclaiming “It is not too much to expect that our children will enjoy in their homes electrical energy too cheap to meter,” among other grand expansions of human wellbeing brought about by nuclear energy.
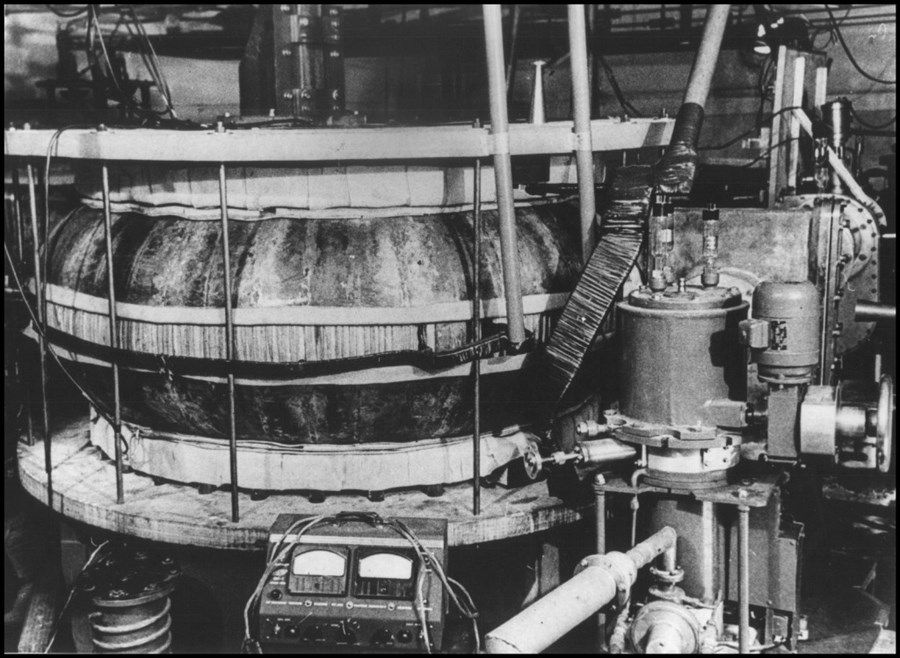
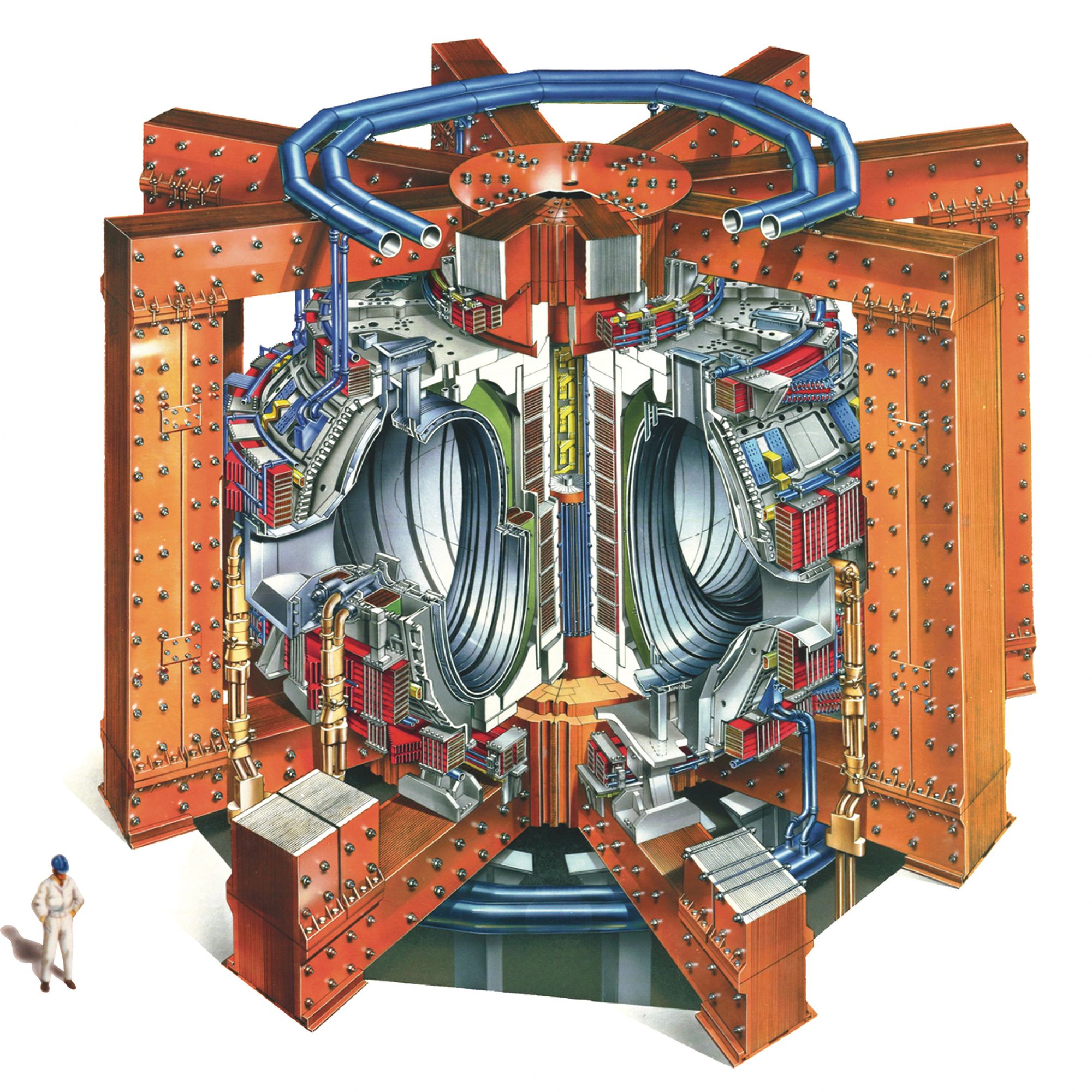
Yet the next few decades of fusion research were ones of slow progress and dead ends, with competing designs for fusion devices and international political rivalries dashing Strauss’ predictions of cheap energy within a generation’s time. The best results came from the Tokamak model, a design from the Soviet Union that used a powerful magnetic field to shape the fusion plasma into a torus with sufficient stability to sustain a reaction. Progress sped up once more starting in 01983, with the establishment of the Joint European Torus, a large Oxfordshire-based reactor that used a modified Tokamak design. The Joint European Torus achieved some of the most efficient results observed before the NIF ignition, achieving a peak ratio of .67 joules of energy produced for every joule consumed.
The NIF, constructed in 02009 in Livermore, a suburb on the eastern edge of the San Francisco Bay Area, opted for a different tack than the magnetic-based Tokamak designs. Whereas those designs derived their power input from strong magnetic fields that confine the fusion torus, the NIF’s Inertial Confinement method instead uses powerful lasers to at once confine and superheat the fusion mass, increasing its temperature and density to induce the fusion reaction. It’s a less energy-efficient approach when accounting for the inefficiency of the lasers themselves, but uses much less tritium fuel and requires the superheating of only a small portion of the mass relative to magnetic methods. Danny Hillis noted in his argument for his 02010 prediction that “the mostly likely way for me to win this bet is with a laser-ignited inertially-confined deuterium/tritium reaction.” NIF’s efforts with inertially-confined reactions have borne fruit: first in a 02021 experiment that achieved a .7 output/input ratio, and then, finally, the December 02022 Ignition event.
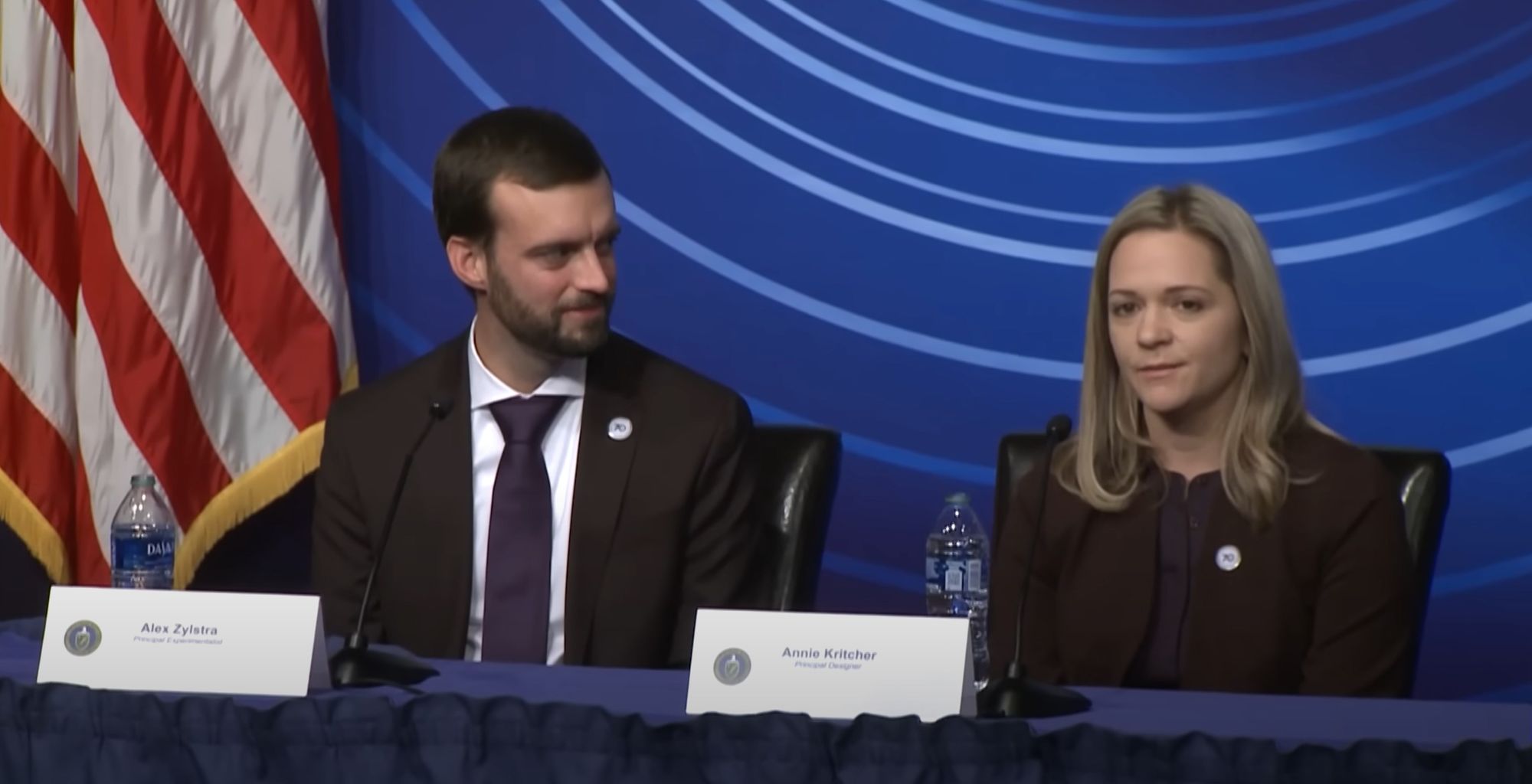
In 02010, Long Now hosted then-NIF Director Ed Moses for a talk in which he proclaimed that the NIF’s laser inertial fusion engine could produce “clean fusion power this decade.” While Moses’ prediction, much like Hillis’, did not quite come to pass, steady progress towards nuclear fusion — and the fast advancement of the past two years — indicates a hopeful future for the technology. In a statement to Long Now Ideas, Hillis noted that “while I often correctly project where technology is heading, I am often surprised how long it takes to get there.” Hillis further reflected “that progress in one area always has to compete with everything that is going on in the world, which almost always slows it down.”
Fusion has taken a long, winding path towards viability, breaking a century’s worth of predictions of its imminent arrival. Even now, it is far from being a feasible option for mass power generation. Yet even at slower than expected rates, research into nuclear fusion continues to progress, keeping the hope of cheap, near-inexhaustible energy alive.